Theme 1: 2016-2019 work
Theme 1, our Earth Systems Modelling work, is developing sophisticated modelling tools to understand and optimize the interaction between enhanced weathering and managed croplands. Read the first (2016-19) of our three-part blog series summarising work since our beginnings in 2016 up to 2023.
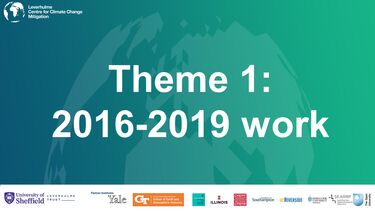
Once you鈥檝e caught up on our 2016-2019 modelling work, don鈥檛 forget to check out the second part, 2019 to 2021 here, and the third part from 2021 to 2023 here.
Jump to:
2016-2017
Advanced Plant-Soil-Mineral Weathering Model
Modelling Global Agricultural Production
2017-2018
Carbon dioxide capture with existing croplands
Hubbard Brook Experimental Forest 鈥 the world鈥檚 first enhanced weathering experiment
Enhanced weathering feedbacks on atmospheric composition and climate
Soil Column Geochemical Model 鈥 a model development tool
2018-2019
Assessing Carbon Dioxide Removal (CDR) Capacity and Costs with Croplands
Modelling Enhanced Weathering Feedbacks on Atmospheric Composition and Climate
Hubbard Brook Experimental Forest 鈥 the World鈥檚 First Catchment-Scale ERW Experiment
2016-2017
Advanced Plant-Soil-Mineral Weathering Model
At the heart of the LC3M's Theme 1 efforts lies the development of an advanced plant-soil-mineral weathering model. This model aims to simulate the intricate interplay between geochemical processes, soil mineral weathering, and agricultural activities. Leveraging a two-pronged strategy, researchers are constructing a detailed single-column soil reactor model and a computationally efficient global weathering model. The former, drawing upon the versatile PHREEQC platform, simulates thermodynamic and kinetic reactions of minerals influenced by root activity, microbial dynamics, and climatic conditions. Notably, the inclusion of silica cycling in this model holds promise for exploring its role in plant defence mechanisms against pests and pathogens. Meanwhile, the latter model, the 91直播 global weathering model (Taylor et al. 2016), scales up these insights to a field-to-regional level, enabling simulations of carbon capture by enhanced weathering in croplands. This agricultural-climate transaction model incorporates essential processes such as nitrogen cycling, necessitating a nuanced treatment of soil nitrogen species interactions. Ongoing development and testing are refining the model's capabilities, marking significant progress in understanding the potential of enhanced weathering as a carbon capture mechanism.
Modelling Global Agricultural Production
Simultaneously, we are enhancing the 91直播 Dynamic Global Vegetation Model (SDGVMv2.0) to encompass agricultural management practices. This updated model aims to capture the complexities of global crop production, soil biogeochemistry, and fertilizer interactions. Through collaborations with Oak Ridge National Laboratory, the model integrates state-of-the-art carbon assimilation schemes and soil carbon dynamics, enabling simulations of daily fluxes of key greenhouse gases. Notably, SDGVMv2.0 incorporates modules tailored to major crop types, enabling simulations of crop phenology, irrigation, and fertilizer applications. Preliminary evaluations demonstrate promising agreement with real-world crop yield data, validating the model's accuracy. Moving forward, the coupling of SDGVMv2.0 with regional-scale weathering models holds potential for estimating carbon capture potential in croplands.
By integrating modelling tools with empirical data and scientific collaborations, we are unravelling the complexities of carbon sequestration in managed ecosystems. As the models evolve and mature, they hold promise for informing policy decisions and shaping sustainable agricultural practices in the fight against climate change.
Theme 1 papers that we published up to June 2017 include:
- Taylor, L. L., Beerling, D. J., Quegan, S., and Banwart, S.A. (2017) . Biology Letters, 13, 20160868. Published 5 April 2017 as part of the mini-series 鈥淓nhanced rock weathering: biological climate change mitigation with co-benefits for food security鈥.
2017-2018
Carbon dioxide capture with existing croplands
Traditionally, estimates for removing CO2 from the atmosphere through ERW focused on tropical forests, overlooking the potential of croplands. However, our Earth systems modelling team recognised the need for a more comprehensive approach. We aimed to quantify the CO2 removal potential of ERW in croplands worldwide, using basaltic rock dust to capture atmospheric CO2.
To achieve this, we developed a sophisticated global empirical modelling framework. This model integrates geochemical calculations and economic considerations to provide spatially explicit solutions. By analysing factors such as cropland area, plant productivity, and energy availability for rock grinding, we identified optimal strategies for maximising CO2 removal through ERW.
Our analysis revealed promising results. Productive croplands in countries like the USA, Brazil, India, China, and some western European countries could collectively sequester approximately 2.5 petagrams (Pg) of CO2 per year. Importantly, this represents a significant fraction (20%) of the post-2050 target needed to limit global warming to 2 degrees Celsius, as agreed upon internationally. This indicates that ERW has the potential to play a crucial role in large-scale carbon capture.
One of the most encouraging aspects of our findings is the economic feasibility of ERW. Our model suggests that implementing ERW strategies would cost less than 1 or 2% of national GDP for these key countries. This demonstrates that ERW could be both effective and affordable, making it an attractive option for policymakers and stakeholders seeking to address climate change.
While our current model provides valuable insights, there is still work to be done. We recognise the need to incorporate feedback mechanisms such as the effects of weathering on soil chemistry and plant health. Moving forward, we plan to refine our model by integrating advanced geochemistry platforms like PHREEQC, ensuring that our modelling outputs remain accurate and robust.
Hubbard Brook Experimental Forest 鈥 the world鈥檚 first enhanced weathering experiment
In October 1999, researchers at HBEF embarked on a catchment-scale experiment that marked the world's first known application of ERW on a terrestrial ecosystem. In that experiment, 3.5 metric tons per hectare of finely ground calcium silicate rock, (~90% wollastonite, CaSiO3), were distributed by helicopter over an 11.8-hectare watershed. Originally designed to investigate the effects of calcium addition on reversing the impacts of acid rain on forest growth, the experiment inadvertently paved the way for ERW research.
Over the course of 15 years, the HBEF team collected pre- and post-treatment stream-water chemistry data. These data provide invaluable insights into the export of inorganic carbon in response to the ERW treatment. By analysing these long-term datasets, we gained a deeper understanding of carbon capture due to the treatment in this catchment.
In a new collaboration with Dr Charles T. Driscoll from Syracuse University, Director of HBEF, Dr Lyla Taylor developed software to calculate CO2 consumption (carbon captured by weathering and exported in streamwater) using the PHREEQC platform using those extensive datasets collected at HBEF as inputs. Preliminary results demonstrate proof-of-concept, showing enhanced CO2 fluxes associated with weathering at Hubbard Brook. Detailed analyses suggest that ERW could lead to even greater effective carbon dioxide reduction if combined with nitrate loss mitigation strategies on farmlands. Lyla presented her findings at the Hubbard Brook Co-operators鈥 Meeting in New Hampshire in July 2018. .
Enhanced weathering feedbacks on atmospheric composition and climate
With the appointment of Dr Maria Val Martin, our team is spearheading efforts to investigate the effects of ERW on trace gas emissions and their implications for atmospheric composition and climate feedbacks.
Nitrous oxide (N2O), one of the gases targeted in Maria鈥檚 team鈥檚 research, is a potent greenhouse gas with a global warming potential hundreds of times higher than that of CO2 over a 100-year period. We have observed through field trials in the USA that ERW can disrupt the nitrogen cycle, prompting us to explore its consequences further. By using advanced modelling tools like the Community Earth System Model (CESM), which encompasses various components of the Earth system, we aim to simulate how ERW influences atmospheric composition and climate feedbacks.
To accurately simulate the effects of ERW, we implemented and refined soil nitrogen flux schemes within the CESM's land model. Additionally, we integrated a new soil pH database to account for changes resulting from ERW applications in agricultural soils. By coupling soil nitrogen species with atmospheric models, we can simulate the impact of ERW-induced emissions on atmospheric composition and climate.
We started to evaluate the performance of our model against real-world observations, including data from field measurements in croplands, satellite-based observations, and detailed emission inventories. Preliminary assessments indicated good agreement between our model and global estimates of soil nitrogen fluxes reported in the literature. Once model development and evaluation were complete, our next step was to conduct simulation experiments to test the hypothesis that ERW in agricultural soils can reduce soil fluxes of N2O and nitrogen oxides (NOx), consequently decreasing tropospheric ozone formation and associated crop damage while reducing the radiative forcing of climate.
This initial research into the effects of ERW on trace gas emissions and atmospheric composition represented a crucial step in understanding the full impact of ERW by employing advanced modelling techniques and rigorous evaluation against observational data.
Soil Column Geochemical Model 鈥 a model development tool
In our ongoing quest to understand the complex dynamics of ERW, Peter Wade developed a powerful tool known as the Soil Column Geochemical Model. This model serves as a bridge between experimental work and advanced simulations, allowing us to delve deeper into the processes occurring within soil columns.
The Soil Column Geochemical Model is a fast-computing 'test-bed' sub-model designed to simulate the weathering of soil minerals and added basaltic minerals. It enables us to evaluate various numerical recipes for weathering calculations, considering factors such as mineralogy and particle sizes over multiple years. This model serves as a crucial development tool, allowing us to explore robust formulations before implementing them in larger-scale global models.
The primary goal of this model is to facilitate a deeper understanding of soil processes and their interactions with added mineral grains. By representing the actions of plants and a range of soil components, including clays, organic matter, and dissolved minerals, the model provides insights into how these factors influence weathering dynamics. Collaborating with LC3M researchers from our experimental science team (Theme 2) ensures that the model is calibrated accurately to real-world conditions.
One of the standout features of the Soil Column Geochemical Model is its integration of the PHREEQC geochemical modelling platform. This powerful tool allows us to simulate the complex interactions between minerals, pore waters, and dissolved atmospheric gases, including carbon dioxide. By incorporating these interactions into our simulations, we gain a more comprehensive understanding of soil processes and their role in carbon capture.
As we continue to refine and calibrate the Soil Column Geochemical Model, we're excited about the possibilities it presents. By providing a detailed understanding of soil dynamics, this model will enhance our ability to predict the impact of ERW on carbon sequestration and climate change mitigation efforts. Through collaboration and ongoing research, we're confident that this model will play a crucial role in advancing our understanding of soil processes and their implications for global carbon cycling.
The Soil Column Geochemical Model represents a step forward in our efforts to understand and harness the power of soil in mitigating climate change. By combining advanced computational techniques with real-world data, this model promises to unlock new insights into soil dynamics and their role in carbon sequestration.
Theme 1 papers we published from July 2017 to June 2018 included:
- Beerling, D. J., Leake, J. R., Long, S. P., Scholes, J. D., Ton, J., Nelson, P. N., Bird, M. I., Kantzas, E., Taylor, L. L., Sarkar, B., Kelland, M., DeLucia, E., Kantola, I., M眉ller, C., Rau, G., and Hansen, J. (2018) . Nature Plants, 4, 138-147. Published 19 February 2018.
2018-2019
Assessing Carbon Dioxide Removal (CDR) Capacity and Costs with Croplands
Euripides, Mark and Rafa have continued their work developing their model. This upgraded model offers a granular understanding of ERW's potential, now with country-level detail, focusing on major economies such as the USA, India, China, and Europe. Unlike previous research primarily centred on tropical forest ecosystems, this model zooms in on agriculture's capacity to capture carbon, paving the way for an effective climate change abatement strategy.
At the heart of this model lies a 1-D vertical reactive transport framework, which simulates rock weathering dynamics within soil profiles. By factoring in changes in weathering rates over time and soil depth, we gain insights into the efficiency of carbon capture. One notable improvement involves a more nuanced approach to calculating weathering rates, considering the log-normal particle size distributions of crushed basalt grains. This enhancement allows for a more accurate representation of the weathering process, crucial for assessing ERW's efficacy.
We have introduced the concept of fractal dimension for surface roughness, enabling a better understanding of the relationship between reacting surface area and basalt mass across different scales, from laboratory experiments to field applications. These methodological refinements contribute to a more comprehensive evaluation of ERW's potential as a scalable CDR strategy.
Initial techno-economic assessments reveal promising results, with nations like China, India, the United States, and Brazil identified as key players capable of contributing significantly to CDR targets. Estimates suggest that these countries could potentially sequester 1-2.5 billion tons of CO2 annually, with extraction costs ranging from approximately $50 to $150 per ton of CO2. Importantly, integrating ERW with existing croplands presents an opportunity to align agricultural practices with climate policy objectives.
However, the full potential of ERW hinges upon overcoming various challenges like political and social barriers, and addressing uncertainties surrounding soil weathering rates and sequestration duration. Additionally, we are exploring alternative sources of silicate materials, such as industrial by-products like slag, and basalt mine overburden, which could potentially reduce the need for establishment of new mines.
This work lays the groundwork for a realistic assessment of ERW's role as a CDR strategy. By quantifying its capacity and costs on a global scale, we are not only advancing scientific understanding but also informing policy decisions. Looking ahead, we are making efforts to develop a next-generation 91直播 Dynamic Global Weathering Model.
Modelling Enhanced Weathering Feedbacks on Atmospheric Composition and Climate
In a collaborative effort with the University of Illinois-Energy Farm, Maria made strides in implementing enhanced weathering techniques within agricultural settings, particularly focusing on corn fields. This involved integrating an empirical parameterisation into the land model (CLM5) of the Earth System Model (CESM), aiming to better understand and leverage the potential of enhanced weathering for climate mitigation.
The empirical scheme, derived from extensive soil N2O and pH measurements conducted during LC3M Energy Farm trials spanning 2016 to 2018, forms the backbone of this endeavour. By analysing these measurements, we developed a function that correlates changes in soil pH with variations in denitrification rates, subsequently influencing soil N2O and NOx fluxes. For instance, an increase in soil pH of 0.25 units translates to significant reductions of approximately 15% in soil N2O emissions and about 25% in soil NOx emissions, mirroring field observations.
At that time, the focus lied on evaluating the performance of this new implementation by comparing model results with actual observations. We designed a series of modelling experiments to quantify the impact of reduced soil N2O and NOx emissions, facilitated by enhanced rock weathering, on critical environmental factors. These included assessing the formation of surface ozone and its potential damage to crops, as well as evaluating the radiative forcing of climate associated with these changes.
Key to understanding the significance of these findings was recognizing the role of soil N2O and NOx emissions in driving atmospheric composition and climate dynamics. Nitrous oxide (N2O) is a potent greenhouse gas, with emissions from agricultural activities contributing significantly to global warming. Similarly, NOx play a crucial role in the formation of surface ozone, a pollutant detrimental to both human health and crop productivity.
Hubbard Brook Experimental Forest 鈥 the World鈥檚 First Catchment-Scale ERW Experiment
Following Dr Lyla Taylor's presentation at the Hubbard Brook Annual Co-operators' Meeting in July 2018, another key figure who was present at that meeting, Professor Peter Groffman, contributed invaluable soil respiration and trace-gas flux data. These data revealed a remarkable reduction in soil respiration rates, particularly at higher elevations within the treated catchment. This decrease not only translated into significant carbon savings but also stimulated increased wood production, marking a significant shift in the forest's carbon cycle dynamics.
The ERW treatment facilitated the enhanced delivery of bicarbonate anions and base cations to the oceans through stream water. However, this process was accompanied by the export of sulphate, nitrate, and organic acids, including dissolved organic carbon (DOC), which partially hindered bicarbonate fluxes. Although the export of DOC and nitrate could potentially lead to elevated downstream CO2 and N2O emissions, their overall impact remained relatively minor compared to the gains achieved through reduced soil respiration and enhanced wood production.
Lyla extended her analysis to evaluate the carbon and financial costs associated with the wollastonite treatment, a crucial step in assessing the feasibility of scaling up similar interventions in acid-rain-impacted forests. A manuscript was submitted to Nature Communications on 13 November 2019 and sent out for review.
The HBEF collaboration is also paving the way for future investigations, including partnerships with Dr Shannon Sterling from Dalhousie University, Nova Scotia. We aim to deepen our understanding of CDR mechanisms and the responses of acidified forests to silicate treatments, charting new paths towards sustainable environmental stewardship.
Theme 1 papers we published in the period July 2018-June 2019 include:
- Arnold, S. R., Lombardozzi, D., Lamarque, J.-F., Richardson, T., Emmons, L. K., Tilmes, S., Sitch, S. A., Folberth, G., Hollaway, M. J., and Val Martin, M. (2018) . Geophysical Research Letters, 45.
- Eufrasio-Espinosa, R. M., and Koh, L. S. C. (2019) . In: Shurpali N., Agarwal A., Srivastava V. (eds) Greenhouse Gas Emissions. Energy, Environment, and Sustainability. Springer, Singapore. Pages 87-109. Published 1 November 2018.
There鈥檚 so much information needed to summarise our 8 years鈥 worth of modelling work that we鈥檝e split it up into three parts. Read part 2 from 2019 to 2021 here, and part 3 from 2021 to 2023 here. And do stay tuned for our (much shorter) annual update from Theme 1鈥檚 work over 2023-2024, coming soon!